<< Back to MOTIFvations Blog Home Page
Discussing Heterochromatin & Phase Separation with Dr. Gary Karpen
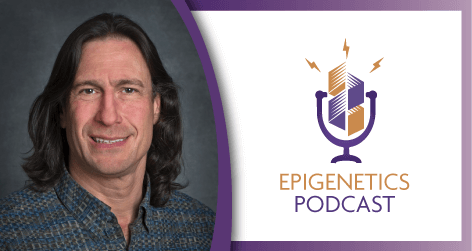
May 28, 2019
Dr. Gary Karpen is currently the director of the Life Sciences Division of the Lawrence Berkeley National Laboratory and an adjunct professor at the University of California, Berkeley. Dr. Karpen is probably most well-known for his work on the structure of chromatin and chromosomes, particularly his work on heterochromatin formation and structure in Drosophila chromosomes.
Stefan Dillinger, the host of Active Motif’s Epigenetics Podcast, recently caught up with Dr. Karpen at the EMBO Workshop on Chromatin and Epigenetics and they chatted about how he got his start in science and the current work in his lab on heterochromatin and phase separation.
Listen to the full interview with Dr. Gary Karpen on Active Motif’s Epigenetics Podcast.
Dr. Gary Karpen Discusses How He Got Started in Science
Dr. Stefan Dillinger: A question that I like to ask this everyone I interview is how did you become interested in biology and start pursuing a career in science?
Dr. Gary Karpen: It was pretty natural for me. I knew in high school that I had an interest in biology and I had a great biology teacher back then. I grew up in suburban New Jersey, in the New York area, and I basically thought that the only thing you did with an interest in biology was medicine. My parents weren't scientists, and we didn't know any scientists, so I didn’t realize science could be a career.
But then when I went to college at Brandeis University and in my sophomore year, I got a job in a Drosophila lab, the lab of Jeff Hall who a couple of years ago got the Nobel Prize, shared with Mike Rosbash and Mike Young, for circadian rhythms. I had absolutely nothing to do with the Nobel Prize-winning research, but it was a great experience and there were a couple of graduate students there, especially Ralph Greenspan, who really encouraged me to think about doing a career in research.
I kept thinking about it, and couldn’t decide between an M.D. and a Ph.D. I couldn't quite let go of that M.D. part, so I decided after college to go and be a technician, and I did that for three years before going to graduate school. And that was also a really great experience.
I wasn't focused on research until after I did it for a couple of years in Jeff Hall's lab. I started out making fly food, I didn't do real research. But by my junior and senior year, I was doing research as well.
My older sister was also an influence on me pursuing a career in science. She is an astrophysicist and I think she probably set the tone for me and my family regarding science, because as I said, neither of my parents were academics, they weren’t scientists.
How Drosophila Became the Model Organism of Choice for Dr. Gary Karpen
Stefan: Drosophila is your model organism of choice, for the most part, why is that? What makes Drosophila a good model for your research?
GK: I learned to love it as an undergrad and then I was a technician in the Schubiger lab working on developmental genetics, that was also working with flies, so I was in fly labs from the beginning. I love genetics and it’s great to have the ability to work with an organism and be able to manipulate it genetically. Now you can do so much more than thirty years ago, but even then, there was the ability to do genetic screens in flies.
This was roughly the time when Janni Nüsslein-Volhard and Erin Wieschaus identified the first segmentation and polarity genes in flies, and Ed Lewis was working on the bithorax complex, so there was a lot of excitement about being able to use genetics to dissect different pathways. But pretty much when I became a graduate student was when I started to become very interested in chromosomes, chromosome structure and function, nuclear structure and function.
What is Heterochromatin & How is it Regulated?
Stefan: Let's just dive into the science you did, meaning heterochromatin. In a 2009 Nature publication you investigated the connection between histone H3K9 methylation and heterochromatin, and also genome stability in Drosophila. What kinds of processes are involved in this methylation and heterochromatin regulation?
GK: We have a slightly different perspective on what heterochromatin is now, but the foundations are the identification of histone H3K9 dimethylation and trimethylation as being enriched in heterochromatic regions.
I should say that when I talk about heterochromatin, I try to be clear about the terminology. Today, most people probably define heterochromatin as pretty much any region in the genome that becomes silenced. There's no right or wrong about the definition of heterochromatin, but I think it’s easy for people to become confused by the terminology they’re using, or the terminology used reflects a certain amount of confusion or lack of knowledge.
For me, I basically go back to the sort of genomic and cytogenetic definition, which is from the early 1920s, Heinz defined heterochromatin as a region of the genome, based on staining with different dyes, that appeared to not decondense during interphase. I don't remember exactly what the dyes were back then, but this was pre-fluorescence. This was still using textile dyes for chromosomes.
We like to talk about the pericentromeric heterochromatin or the peri-telomeric heterochromatin. These are regions that are enriched for repetitive sequences, either simple satellite sequences like ATAT, AGAG, transposons, and transposon fragments. It makes it simpler, for me at least, to think in terms of the genomic definition. We're talking about these particular regions on all the chromosomes.
Stefan: When you look at the definition of chromatin nowadays you have constitutive heterochromatin, and facultative heterochromatin, does your research focus primarily on the constitutive heterochromatin?
GK: Yes, we primarily focus on constitutive chromatin. I should point out that there are blocks of H3K9 methylation that interact with a reader protein, Heterochromatin Protein 1 (HP1), and in turn that can interact with the methyltransferases such as suppressor of variegation 3-9 [Su(var)3-9] or G9A, et cetera, et cetera.
Stefan: Would you consider H3K9 trimethylation as the most defining part of heterochromatin? Or is it the connection between the repetitive elements and the histone marks like H3K9me3?
GK: Everything works together, it's all one big hairball. H3K9 methylation is one of many interactions that can create three-dimensional repressive domains in the nucleus. So that's a distinction from just talking about the DNA and the nucleosomes, that heterochromatin is also an integral part of these nuclear bodies, or nuclear domains, that occupy three-dimensional space – take up volume in the nuclear compartment. That is an important part of heterochromatin function, we think, although there's no definitive evidence for that yet.
H3K9 methylation and the interaction of these modified histones with HP1 is one of many interactions important for forming these nuclear bodies. HP1 also interacts with many, many other proteins and probably RNA to help form these domains.
Stefan: How do you think about the repetitive elements, like those that you just mentioned? They don’t seem to have a clear function. They do not code for proteins or any other things, so what do they do, and why do we still have them if they don't have the distinct function that we see in other regions of the genome?
GK: There are in fact essential protein-coding genes in heterochromatin. For example, in Drosophila there's the only copy of RAD21, a cohesin component, and it is actually embedded in heterochromatin. It's somewhat of a mystery that these genes are expressed despite having high levels of K9 methylation and HP1.
But in general, heterochromatin seems to be more important for chromosome functions like segregation; the centromeres are embedded inside heterochromatin. Other aspects of nuclear architecture are also impacted by heterochromatin as well, such as meiotic. So it's a complementary or different kind of function compared to protein coding. There is more evidence emerging that the simple satellite sequences, their transcription or transcripts, also seem to be regulated by heterochromatin.
How Do Proteins Access Heterochromatin Regions & How Does Heterochromatin Regulate DNA Repair and Recombination?
Stefan: Since heterochromatin is such a densely packed region of the nucleus that excludes most proteins, so how do proteins like DNA repair factors access the heterochromatin? How do they get there and how do they access then DNA?
GK: The issue about exclusion is something that we're reassessing. The classical view has been that it's a highly compacted state, in a sense a brick wall, that proteins can't access. But of course they can. Many, many proteins do access the heterochromatin and that's likely to be because it's a very dynamic domain. It's not a brick wall, it's constantly in motion, the molecules within it are constantly in motion.
So, with respect to DNA repair, what was shocking or striking about that, and this was the work of Irene Chiolo, a postdoc at the time, was that the double-strand breaks would actually start DNA repair inside the heterochromatin domain. They would do all the initial stages of DNA repair. They would be recognized as double-strand breaks, you get phosphorylation of H2A variants, and you do resection, and you recruit all the single-strand binding proteins, except you couldn't recruit RAD51 or its loading factor, BRCA2. It turns out that you do load them, but only after the double-strand breaks leave the heterochromatin domain and ultimately end up at the nuclear periphery.
Stefan: Meaning that the heterochromatic region that is repaired becomes more euchromatic, in a sense? Or is it still different than repairing euchromatic regions?
GK: Well, we do think that, from later work involving a histone demethylase, that locally they become more euchromatic-like, or they go to a ground state that is more euchromatic-like.
But to me, the interesting thing is why this mechanism is in place. This is not the way that double-strand breaks have to be repaired if they're in the euchromatin. The hypothesis that we proposed is that when you have a high concentration of these very simple repeats, and there are millions of copies of them in this one place, the last thing you want to be doing is to have a receptive RAD51-coated filament ready and able to do homology search but not able to do recombination.
In fact, when you remove the heterochromatin proteins, that's exactly what you see. You remove HP1 or Su(var)3-9, you get massive recombination between repeats in these domains and they can't even segregate out during cell division.
The idea is that you separate, in space and in time, the initial stages of homologous recombination repair, like resection, but you don't allow them to be coated with the protein RAD51 and associated factors that will promote homologous recombination or complete it, until you're outside the domain, away from all those other repeats, and associated with either your sister or your homolog.
We, in fact, later showed that single-break systems, through the work of Aniek Janssen, that they can associate with their homolog and repair off of their homolog.
You want to avoid recombination with these regions of the genome that contain so many repeats. This goes back to your question from earlier, why are they there? Why are these repetitive elements conserved in evolution if they are so problematic? If you have a double-strand break in a normal chromosome in the heterochromatin regions and it recombines with another heterochromatin region, you create dicentric chromosomes (that have two centromeres), or you create acentric chromosomes, and the genome stability becomes highly unstable.
It seems like heterochromatin proteins, and probably RNAs, have evolved mechanisms to repress that and one of these mechanisms is separating the early stages of homologous repair from the later stages in time and space.
Stefan: You mentioned that spatial separation is important for the heterochromatin-mediated regulation of recombination and DNA repair. How does chromatin move within the nucleus, what is the mechanism?
GK: Well, recently Irene Chiolo, who is now at a USC in her own position, published a paper in Nature showing that active myosin complexes help promote movement.
We think that that's what happens after they leave the domain, so there's no evidence that active myosin-mediated movements are responsible for taking them out of the domain. We think that leaving these nuclear domains is actually related to phase separation and what you mentioned before, which is at double-strand breaks you become more euchromatic-like. In fact, if you lose the association with HP1 or lose K9 methylation that you will, just because of the energetics of the system, favor leaving the domain rather than staying in.
Stefan: So, it's more biophysics that leads to this separation, and then when it's no longer associated with heterochromatin then it moves more easily, to be then repaired because it's easier to repair somewhere else?
GK: But it's also mediated by the factors that get recruited to the double-strand breaks, including relatives of condensins and cohesins called Smc56 and their associated factors. There's a SUMO ligase and a ubiquitin ligase, they sort of form a chain of associations that lead them to associate with the SUMO-targeted ubiquitin ligase (STUbL) locus at the periphery.
Stefan: You also had a publication recently focusing on this and you identified a histone demethylase having a role in this process. Can you just comment on that a little bit?
GK: Yeah, this was work Aniek Janssen, who has a position at Utrecht University, and Serafin Colmenares. Basically, Serafin had previously shown that KDM4A is a histone demethylase, it clearly does histone H3K36, it might do H3K9, and it might do H3K56. We and others have had a lot of problems demonstrating what is direct and what is indirect in terms of the demethylation activity.
Regardless, what they found was that KDM4A was required only for heterochromatic double-strand break repair. There no defects in the timing or the type of repair in euchromatic double-strand breaks when you get rid of KDM4A. This was all done with single-break systems, not irradiation, that Aniek developed.
However, the heterochromatic breaks would take five times as long to be repaired and we saw a change in the relative frequency, of non-homologous end joining versus homologous recombination. Normally, there's more non-homologous end joining than homologous recombination, but it's the same for heterochromatic and euchromatic breaks in the fly system. In the absence of KDM4A, you have more homologous recombination than non-homologous end joining.
Stefan: Would that also mean that those regions get a different chromatin signature?
GK: We did look at that, and it's very difficult because of the timing. You can't really induce breaks and immediately be able to assess histone modification. But yes, what we saw was a change, loss of H3K56 methylation, which is another heterochromatic-associated mark, loss of the H3K9 methylation at normal breaks and then retention of those.
The idea is that you take heterochromatic breaks and you make them look more like euchromatic breaks, but locally. We think, though we have not proven, that that's what leads to it; the double-strand breaks leaving the domain, where they then get latched on to the active myosin system.
Something we're following up on is that the histone methylation state affects how it gets repaired, whether it's by homologous recombination or by non-homologous recombination. This has potential use in genome engineering and especially for engineering difficult regions of the genome, but we haven't demonstrated that directly yet.
Prefer listening over reading? Check out the full interview with Dr. Gary Karpen on Active Motif’s Epigenetics Podcast.
Phase Separation & Its Role in Heterochromatin Formation
Stefan: A term that now came up relatively often, during our interview now, is phase separation. You published a paper in 2017 in Nature and this was called “Phase Separation Drives Heterochromatin Domain Formation.” What do you mean by phase separation in this context, and how does it work?
GK: When thinking about the “liquids” in the nucleus, it’s important to remember that not all liquids are alike. The key to being a liquid is that you have to have mobility of molecules. Basically, the idea is that you need to be able to contact other molecules, many other molecules, and these need to be weak interactions. A strong interaction will be a solid, will end up being the solid state of matter. So, you need to have all these molecules that are weakly interacting and they have to have an affinity for one another. Considering heterochromatin, it's not just about HP1, it's about all the other things it interacts with. There are hundreds of interaction partners. With H3K9 methylation as well.
When you have different liquids, what makes them able to fuse is the fact that they have similar compositions of interactions with similar interaction strengths, and when they come together, they can mix together and continue this process of touching each other and making sure that they're in the right place or associated with the right factors.
But if you take, for example, a polycomb body and you try to fuse it with an HP1 body, those two sets of molecules do not have the desire, ability, to interact with each other weakly. This is essentially phase separation.
I think that is a very exciting area that's yet to be explored, as to exactly what it is that makes these things distinct. If we didn't have that, then everything would just mix together in the nucleus and we wouldn't have separate bodies. That would probably not be a good thing.
What I think is very clear is that phase separation plays a critical role in nuclear organization. There are many ideas that still need to test directly, experimentally in vivo, but I'm personally convinced that that's true, at least for heterochromatin.
But what's unclear is whether there's a direct role for phase separation in functions of the genome, other than the organization. The organization itself could be important, and could concentrate molecules in a way that's required for function, but is there some aspect of the biophysical properties of a body, what distinguishes the polycomb body from the HP1 body, and both of those from the super-enhancer bodies? Is it material differences? More liquid, less liquid? Compositional differences. Viscosity. All these sorts of things. We're trying to investigate more on the functional side, and this expressly focused on in vivo.
Stefan: That's a good place for my last question because as we just sort of took a journey through your scientific career, can you maybe give a short summary of what you would consider as your most important finding or most stunning scientific finding in your career? And what would you like to do in the future?
GK: I would say there are two. We didn't talk about centromeres, but many years ago work that we did along with Robin Allshire working in S. pombe, we discovered that what mediates centromere identity and propagation is an epigenetic mechanism. Not a genetic mechanism or sequence-based mechanism like what was previously demonstrated in Saccharomyces cerevisiae. That was a very exciting time and that led to a lot of very interesting studies, both within my lab and especially outside of my lab.
I would say that the phase separation work is also very exciting because of all the implications that it has for genome architecture, for example diffusion, how does that regulate why and how things are held together in space. What's exciting about it to me is that there are so many opportunities for regulation just by phosphorylating one residue or dephosphorylating a residue to form these bodies, to dissolve them, and so, what we're now and what I see us doing for the foreseeable future, is to try to ask the question about function of the genome and to get down into the specifics of how that works and what the impact is.
Stefan: Gary, thank you very much for your time, this was a fascinating conversation and discussion!
GK: Thank you. It was fun.
Check out the full interview with Dr. Gary Karpen on Active Motif’s Epigenetics Podcast to learn more about his views on what heterochromatin is and how it actively regulates biological processes, the role and importance of phase separation, and more.
<< Back to MOTIFvations Blog Home Page